Additively manufactured energetic materials (AMEMs) are highly loaded polymer composites within some curable binder. In order to investigate the high strain rate mechanical response and the shock propagation characteristics, simulant materials are being developed and printed. Simulant materials are chosen to mimic chemistry, morphology, and printability of actual energetic materials. Additive manufacturing via pneumatic direct write extrusion offers the ability to directly choose the shape, size, and other characteristics of these simulant highly-loaded polymer composites. The additive process, however useful, inherently introduces heterogeneities such as particle aggregation within a filament, and porosity, periodicity, and anisotropy across various length scales of the material. These can occur within filaments, between filaments, and across the whole of a layer or the whole sample. Heterogeneities such as these can affect high strain rate mechanical properties in difficult to predict ways that can prevent standardization of properties.
This research project plans to investigate the influence of process-induced structural heterogeneities and anisotropy on high strain rate mechanical response of simulant AMEMs. Firstly, the effect of printing parameters on microstructure at several length scales will be investigated by quantitative microstructural characterization. By imaging with X-Ray Computed MicroTomography (uCT), non-destructive imaging can be performed on whole samples or sections of samples. This imaging technique allows for various resolutions of interest, down to the tens of microns, in order to image the heterogeneities. Microstructural features will be investigated with traditional quantitative stereology techniques as well as with 2-point correlation functions. By quantitatively characterizing the microstructure, a mathematical representation of the distributions and scales of heterogeneities will be created. An example of a uCT image of a mock-AMEM is shown below.
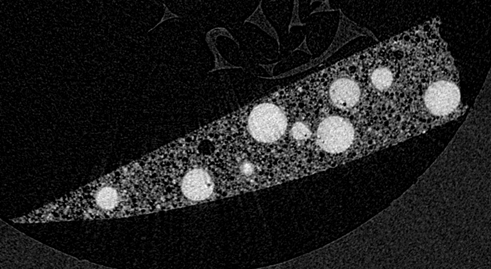
Figure 1. Micro-CT Image of a mock-AMEM.
In addition to the quantitative characterization of these AMEMs, a novel sensor utilizing quantum dots will be developed. Quantum dots (QDs) are nanoscale semiconductors with size-dependent characteristic emissions in the visible and near-infrared range due to quantum confinement effects. In recent work, Kang et al. [1] and Xiao et al. [2] have shown that QDs exhibit unique physical and optical properties under compression, which position them as possible mesoscale pressure sensors for shock-compression studies. We will investigate various QDs as optomechanical sensors to monitor pressure distributions during shock-compression. Emissive QDs of various shapes (spherical, rod-like, tetrapods), compositions, and architectures synthesized at Georgia Tech will be subsequently investigated for use as sensors to monitor the shock-compression response of materials. The shock-compression behavior of heterogeneous particle-filled composites, such as mock-AMEMs, results in complex interactions, which makes it difficult to predict their energetic and mechanical response. Their behavior is often dominated by the mesoscale defects (including porosity) or interactions of the shock wave with interfaces and particulates. Traditional diagnostic methods, such as velocity interferometry, enable good temporal measurements, but limit the spatial resolution necessary to provide sufficient information regarding mesoscale processes that influence the performance. Measurements with interferometry are averaged over some spatial area (on the millimeter scale) and give surface information only. Hence, the goal is to investigate the use of quantum dots as optomechanical sensors to determine mesoscale pressure distributions in composite structures.
References
[1] Kang, Z., Banishev, A., Lee, G., Scripka, D., Breidenich, J., Xiao, P., Christensen, J., Zhou, M., Summers, C., Dlott, D. and Thadhani, N. (2016). Journal of Applied Physics, 120(4), p.043107.
[2] Xiao, P., Ke, F., Bai, Y. and Zhou, M. (2017). Composites Part B: Engineering, 120, pp.54-62.